Research Progress of Diabetic Tubulopathy
-
摘要: 糖尿病肾病已成为慢性肾脏疾病和终末期肾病的主要原因。更好地了解糖尿病肾病早期变化的特征和分子机制,有助于开发预防该慢性病变的新策略。早期糖尿病肾病损伤机制和治疗靶点的研究已由以肾小球损伤为中心转移到糖尿病肾小管病(diabetic tubulopathy,DT)。就血糖、补体、生物标志物和线粒体在糖尿病肾小管病的作用机制研究和相关治疗靶点进行综述,以期为早期糖尿病肾病的诊断和治疗提供新的方向。Abstract: Diabetic kidney disease(DKD) has become a major cause of chronic kidney disease and end-stage renal disease. A better understanding of the characteristics and molecular mechanisms of the early changes in DKD will contribute to the development of new strategies to prevent this chronic disease. The research on the damage mechanism and therapeutic targets of early DKD has shifted from glomerular damage to diabetic tubulopathy(DT). This article summarizes the mechanism and therapeutic targets of blood glucose, complement, biomarkers and mitochondria in DT, with the purpose to provide a new direction for the diagnosis and treatment of early DKD.
-
Key words:
- Diabetic kidney disease /
- Diabetic tubulopathy /
- Complement /
- Biomarker /
- Mitochondria
-
近年来,随着对氧化还原类蛋白质翻译后修饰的深入研究,蛋白质巯基亚硝基化在细胞信号转导调控中的作用日益受到人们关注。病理状态下,由诱导型一氧化氮合酶(inducible nitric oxide synthase,iNOS)表达产生的高浓度一氧化氮(nitric oxide,NO)可引起区域相关蛋白质S-亚硝基化修饰,影响多种疾病的病理生理过程。研究表明,蛋白质S-亚硝基化在癌症的发生发展中扮演着重要作用,本文主要就其与消化道肿瘤相关进展作一综述。
1. NO的S-亚硝基化作用
NO是一种存在于细胞内和细胞外的信使,它可以通过介导靶细胞中的多种信号传导途径从而调节多种细胞功能,如免疫反应,炎症反应,细胞凋亡、增殖,神经信号传递等[1]。在哺乳动物细胞中,NO的主要来源是由三种亚型的一氧化氮合酶(nitric oxide synthase,NOS)所介导,分别是依赖经典cGMP信号通路的Ⅰ型神经元型一氧化氮合酶(neuronal nitric oxide synthase,nNOS)和Ⅲ型内皮型一氧化氮合酶(endothelial nitric oxide synthase,eNOS),而Ⅱ型诱导型一氧化氮合酶(iNOS)则是将炎症与癌症联系起来的关键作用酶。大量实验研究和基于人群的研究表明,慢性炎症在致癌中的作用越来越明显,特别是在癌促发和进展阶段[2-3]。例如肝、胆、胃、前列腺部癌和胰腺癌都与炎症有因果关系。炎症可诱导多种转录因子的异常激活,例如核因子-κB(nuclear factor-k-gene binding,NF-κB),激活蛋白1(activator protein-1,AP-1)和缺氧诱导因子-1α(hypoxia inducible factor 1,HIF-1α)等。iNOS是炎症的主要介质,它不依赖钙离子信号,在细胞静息状态下不表达或很少表达,在炎症或免疫反应过程中会通过巨噬细胞和上皮细胞释放大量NO,受炎症因子刺激或细胞应激后活化产生大量NO,NO进而氧化形成活性氮(reactive nitrogen species,RNS),通过诱导蛋白质翻译后S-亚硝基化修饰作用影响炎症和细胞凋亡[4]。细胞内高浓度的RNS会导致高浓度的S-亚硝基化蛋白,这个过程可以通过改变癌症发展过程中重要的靶标和途径来驱动癌变,其速度比健康组织要快的多[5]。相等浓度的NO和超氧化物会导致ONOO-的形成,而过量2至3倍的NO主要会导致三氧化二氮(N2O3)的形成,这被认为是细胞内微环境中的主要S-亚硝基化剂[4]。在已知的相关研究中,S-亚硝基化底物的数量达到3 000 多种,包括多种转录因子、代谢酶、氧化还原酶、蛋白激酶、离子通道、转运蛋白等,而其对底物特异性的因素之一就是NOS能与作为S-亚硝基化目标的蛋白质之间的相互作用[6]。
2. S-亚硝基化与消化系统肿瘤
2.1 胃癌
尽管胃癌的治疗在外科和新辅助化疗和单纯化疗方面取得了进展,但胃癌仍然是全球卫生系统的主要负担。炎症与癌症是相互作用的病理生理过程,胃癌发展的主要危险因素之一是幽门螺杆菌的感染,尽管胃黏膜会产生强烈的免疫反应,但它仍能存活下来,从而有利于从慢性胃炎到腺癌等疾病。沉默信息调节因子1(silent information regulator transcript 1,SIRT1)是一种依赖烟酰胺腺嘌呤二核苷酸(nicotinamide adenine dinucleotide,NAD+)辅酶的III型组蛋白去乙酰化酶,通过使细胞凋亡,DNA损伤修复以及代谢相关的各种蛋白质去乙酰化,成为各种病理生理过程的重要调节剂[7]。一方面,SIRT1可通过调节多种组蛋白去乙酰化以调节染色质功能,如组蛋白4赖氨酸4; 另一方面,SIRT1可通过去乙酰化作用调节非组蛋白生成,如转录因子p53、NF-κB、叉头框蛋白O(forkhead box protein O,FOXO)等,从而调控基因的表达[8-11]。SIRT1的过表达通常与不良预后相关[12]。胃上皮细胞暴露在幽门螺旋杆菌环境下会导致iNOS的产生,进而可能导致胃癌的基因改变,实验和临床证据表明,在胃癌患者第一次的内镜检查中显示出会有较高的iNOS表达[13]。SIRT1的S-亚硝基化作用,可通过iNOS调节合成的NO作用实现。亚硝基化的甘油醛-3-磷酸脱氢酶能够被转运到细胞核内与SIRT1相互作用,在一氧化氮合成酶的作用下将亚硝基传给SIRT1,使SIRT1的第387和390位半胱氨酸位点发生亚硝基化[14]。证据表明SIRT1激活剂作用的细胞表现为炎症反应减轻[15]。NF-κB是一种普遍存在的转录因子,主要由p65/p50亚基组成,对人类炎症和各种恶性肿瘤的发生起着至关重要的作用[16]。SIRT1的S-亚硝基化修饰可以抑制SIRT1活性,进而激活NF-κB[17]。肿瘤坏死因子α(tumor necrosis factor α,TNF-α)是具有多功能的炎症细胞因子,通过与受体相互作用,激活NF-κB,TNF-α诱导下游SIRT1蛋白与NF-κB的RelA/p65亚基相互作用,并通过在赖氨酸310处使RelA/p65去乙酰化来抑制转录[18-19]。用非甾体抗炎药治疗胃溃疡应激模型,在体内 S-亚硝基化 NF-κB p65 蛋白,抑制胃粘膜前列腺素E2(Prostaglandin E2,PGE2),显著增加了促炎细胞因子TNF-α水平。根据这些发现,可以得出结论:SIRT1抑制促炎信号传导,而这种信号通常在转化的癌细胞中被不适当地激活。因此,可以通过抑制异常激活的促炎信号来预防炎症相关的癌变。遗憾的是,目前缺乏相关蛋白质S-亚硝基化与胃癌发展的相关研究,有待进一步探究。
2.2 结直肠癌
一项纳入19例结直肠癌患者的临床研究中结果显示癌组织中的总S-亚硝基化水平显著高于相邻的正常组织[20]。在肠道中,iNOS活性的增加被认为是促炎的[21-22]。6-丙酮酰四氢蝶呤合成酶(6-pyruvoyl tetrahydropterin synthase deficiency,PTPS)是参与从头合成iNOS的必要辅助因子,在早期结直肠癌中高表达的 PTPS 在低氧条件下被腺苷酸活化蛋白激酶(AMP-activated protein kinase,AMPK)在 Thr 58 磷酸化,这种磷酸化促进PTPS与转化生长因子结合蛋白1(latent transforming growth factor β binding protein 1,LTBP1)的结合,并随后通过PTPS / iNOS / LTBP1 复合物中近端偶联 BH4 的产生来促进 iNOS 介导的 LTBP1 S-亚硝基化,LTBP1 S-亚硝基化导致蛋白酶体依赖性 LTBP1 蛋白降解,阻止了转化生长因子-β(transforming growth factor-β,TGF-β)的分泌和TGF-β诱导的肿瘤细胞生长停滞,并促进了早期结直肠肿瘤的发展[23]。当使用iNOS抑制剂1400 w时作用于结直肠癌细胞HT29和HCT116时,表现为抑制细胞增殖和迁移,同时使用1400 w和5氟尿嘧啶(5-fluorouracil,5-FU)能提高抗癌的增敏性[24]。有趣的是,在结直肠癌中,并非所有蛋白质的S-亚硝基化都能促进肿瘤的生长。微囊藻毒素-LR (microcystin-LR,MC-LR) 是一种蓝藻毒素,可启动正常细胞和肿瘤细胞的凋亡,当使用MC-LR处理结直肠癌SW480细胞72 h后,NO应激诱导糖酵解酶3-磷酸甘油醛脱氢酶(glyceraldehyde-3-phosphate dehydrogenase,GAPDH)Cys150位点的 S-亚硝基化修饰,导致其与泛素连接酶 Siah1 结合后的核易位,核 GAPDH 可以促进核靶蛋白的降解,启动p53诱导的细胞死亡[25]。同样的研究结果,NO供体或iNOS过表达诱导结直肠癌细胞质中促凋亡刺激物死亡受体CD95的Cys199和Cys304的S-亚硝基化,促使肿瘤坏死因子受体Fas重新分布到脂筏,形成诱导死亡信号复合物,促进了结肠癌细胞的死亡,同时也发现Fas在对细胞凋亡不太敏感的结直肠癌细胞中的表达量通常比较低,因此Fas S-亚硝基化诱导剂能提高Fas的敏感性[26]。不同研究结果表明,在结直肠癌中,不同蛋白质的S-亚硝基化可以通过不同的通路促进或抑制癌症的发展。
2.3 肝细胞癌
人类的肝细胞癌(hepatocellular carcinoma,HCC)与iNOS的升高表达密切相关,S-亚硝基化的应激性增加促进了HCC的发生和进展。过去的研究中已经证明酒精和慢性肝炎是HCC的危险因素,实验研究发现,酒精喂养的小鼠蛋白质S-亚硝基化增加进而诱导HCC形成[27]。主要的脱亚硝基化酶,S-亚硝基谷胱甘肽还原酶(glutathione reductase,GSNOR)是一种无处不在的高度保守的酶,它的下调导致 S-亚硝基化蛋白的积累,可以防止过度的蛋白质S-亚硝基化,具有线粒体自噬降解能力,这与肿瘤的诱导和进展有关[28-31]。值得注意的是,GSNOR在大约50%的HCC患者中显著减少,在GSNOR−/−的小鼠极易感染和引发HCC[32]。在缺失GSNOR的小鼠经iNOS诱导后,可以致Parkin蛋白S-亚硝基化增加,进而促进肝癌的发展,可以通过iNOS抑制剂来预防由GSNOR缺乏引起的HCC[32-33]。在用一线靶向药索拉菲尼治疗HCC晚期患者时,检测到凋亡诱导受体Fas的S-亚硝基化降低,这也有助于减少肝癌细胞的生长[34]。同样的结果,O6-烷基鸟嘌呤-DNA烷基转移酶(O6-alkylguanine-DNA alkyltransferase,AGT)是参与 DNA 损伤反应的关键酶,在体外和体内均检测到AGT Cys145 处的 S-亚硝基化,促进了AGT 蛋白酶体降解以及致癌烷基鸟嘌呤的积累,从而促进HCC的发展[35]。但是,在HCC中,是否不同蛋白质的S-亚硝基化都具有促癌作用仍需进一步验证。
2.4 胰腺导管腺癌
胰腺导管腺癌(pancreatic ductal adenocarcinoma,PDAC)源于胰腺外分泌细胞,是最常见的胰腺癌亚型,占胰腺恶性病例的85%以上[36]。PDAC中iNOS、eNOS和nNOS的表达明显增加,在这项研究中,通过特定部位的蛋白质组学,在PDAC患者癌组织和胰腺导管腺癌PANC-1细胞中发现的S-亚硝基化蛋白数量比相邻的非癌组织多,还表明在相邻的非癌组织中,S-亚硝基化蛋白主要与基本生物过程相关,如原细胞代谢、生物质量调节、应激和刺激反应、催化过程、氧化和减少、继发代谢和转化启动,但在PDAC组织和PANC-1细胞中,S-亚硝基化蛋白在细胞周期、细胞分裂、细胞运动等癌症的启动、发展和转移过程中扮演着重要的角色,这些过程都与肿瘤形成有关[37]。在进行KEGG通路数据库分析表明,胰腺癌通路的成分被广泛S-亚硝基化,如细胞分裂周期蛋白42(cell division cycle 42,CDC42),转录激活因子1(activator of transcription 1,STAT1), Ras相关C3肉毒菌素物底物1(ras-related C3 botulinum toxin,Rac1),Ras相关C3肉毒菌素物底物2(ras-related C3 botulinum toxin,Rac2)和信号传导与转录激活因子3(activator of transcription 3,STAT3)[37]。此外,NOS抑制剂显著抑制了PANC-1细胞中的STAT3 S-硝基化,导致STAT3磷酸化和PANC-1细胞存活率显著提高,表明蛋白质S-亚硝基化在PDAC发育中的重要作用[37]。在这项研究中,STAT3被确定为胰腺癌背景下的S-亚硝基化蛋白,NOS抑制剂STAT3 S-硝基化减少,STAT3磷酸化和胰腺癌细胞存活率升高,进一步表明S-亚硝基化在PDAC发病机理中的重要作用[37]。在PDAC中,蛋白质的S-亚硝基化可以促进癌细胞的增殖。
3. 小结
综上所述,各种证据表明,蛋白质的S-亚硝基化广泛参与了消化道系统肿瘤的发生发展与耐药过程,不同蛋白质在S-亚硝基化后会发生功能获得或功能丧失的修饰,与肿瘤的发生发展以及耐药相关。考虑在疾病炎症阶段检测不同癌症蛋白质表达,干预其S-亚硝基化状态,会更有利于降低癌症的发生率,对于已经发展为肿瘤的患者对相关蛋白S-亚硝基化修饰进行干预,为肿瘤治疗提供新的靶点并且可以延缓患者对肿瘤药物的耐药性。尽管如此,考虑到S-亚硝基化在不同癌症类型中的众多靶点,且目前相关研究并不多,用于操纵该过程以获得治疗效果的方法有很多种,探索蛋白质S-亚硝基化在不同肿瘤微环境中不尽相同的作用使其具体分子机制仍待深入探讨,有望为癌症患者的临床治疗及预防开辟新路径。
-
[1] Fineberg D,Jandeleit-Dahm K A,Cooper M E. Diabetic nephropathy: Diagnosis and treatment[J]. Nat Rev Endocrinol,2013,9(12):713-723. doi: 10.1038/nrendo.2013.184 [2] Huang S,Xu Y,Ge X,et al. Long noncoding RNA NEAT1 accelerates the proliferation and fibrosis in diabetic nephropathy through activating Akt/mTOR signaling pathway[J]. J Cell Physiol,2019,234(7):11200-11207. doi: 10.1002/jcp.27770 [3] Alicic R Z,Rooney M T,Tuttle K R. Diabetic kidney disease: Challenges,progress,and possibilities[J]. Clin J Am Soc Nephrol,2017,12(12):2032-2045. doi: 10.2215/CJN.11491116 [4] Yamanouchi M,Furuichi K,Hoshino J,et al. Nonproteinuric versus proteinuric phenotypes in diabetic kidney disease: A propensity score-matched analysis of a nationwide,biopsy-based cohort study[J]. Diabetes Care,2019,42(5):891-902. doi: 10.2337/dc18-1320 [5] Pichaiwong W,Homsuwan W,Leelahavanichkul A. The prevalence of normoalbuminuria and renal impairment in type 2 diabetes mellitus[J]. Clin Nephrol,2019,92(2):73-80. doi: 10.5414/CN109606 [6] Lamacchia O,Viazzi F,Fioretto P,et al. Normoalbuminuric kidney impairment in patients with T1DM: Insights from annals initiative[J]. Diabetol Metab Syndr,2018,10(60):1-8. [7] Chen J,Wang X,He Q,et al. YAP activation in renal proximal tubule cells drives diabetic renal interstitial fibrogenesis[J]. Diabetes,2020,69(11):2446-2457. doi: 10.2337/db20-0579 [8] Zeni L,Norden A G W,Cancarini G,et al. A more tubulocentric view of diabetic kidney disease[J]. J Nephrol,2017,30(6):701-717. doi: 10.1007/s40620-017-0423-9 [9] Haraguchi R,Kohara Y,Matsubayashi K,et al. New insights into the pathogenesis of diabetic nephropathy: Proximal renal tubules are primary target of oxidative stress in diabetic kidney[J]. Acta Histochem Cytochem,2020,53(2):21-31. doi: 10.1267/ahc.20008 [10] Phillips A O,Steadman R,Morrisey K,et al. Exposure of human renal proximal tubular cells to glucose leads to accumulation of type IV collagen and fibronectin by decreased degradation[J]. Kidney Int,1997,52(4):973-984. doi: 10.1038/ki.1997.419 [11] Morrisey K,Steadman R,Williams J D,et al. Renal proximal tubular cell fibronectin accumulation in response to glucose is polyol pathway dependent[J]. Kidney Int,1999,55(6):2548-2572. [12] Perkovic V,Jardine M J,Neal B,et al. Canagliflozin and renal outcomes in type 2 diabetes and nephropathy[J]. N Engl J Med,2019,380(24):2295-2306. doi: 10.1056/NEJMoa1811744 [13] Neuen B L,Young T,Heerspink H J L,et al. SGLT2 inhibitors for the prevention of kidney failure in patients with type 2 diabetes: A systematic review and meta-analysis[J]. Lancet Diabetes Endocrinol,2019,7(11):845-854. doi: 10.1016/S2213-8587(19)30256-6 [14] Cherney D Z I,Zinman B,Inzucchi S E,et al. Effects of empagliflozin on the urinary albumin-to-creatinine ratio in patients with type 2 diabetes and established cardiovascular disease: An exploratory analysis from the EMPA-REG OUTCOME randomised,placebo-controlled trial[J]. Lancet Diabetes Endocrinol,2017,5(8):610-621. doi: 10.1016/S2213-8587(17)30182-1 [15] Yu S M,Leventhal J S,Cravedi P. Totally tubular,dude: Rethinking DKD pathogenesis in the wake of SGLT2i data[J]. J Nephrol,2021,34(3):629-631. doi: 10.1007/s40620-020-00868-0 [16] Lans H,Hoeijmakers J H. Genome stability,progressive kidney failure and aging[J]. Nat Genet,2012,44(8):836-838. doi: 10.1038/ng.2363 [17] Daroux M,Prevost G,Maillard-Lefebvre H,et al. Advanced glycation end-products: Implications for diabetic and non-diabetic nephropathies[J]. Diabetes Metab,2010,36(1):1-10. doi: 10.1016/j.diabet.2009.06.005 [18] Kumar Pasupulati A,Chitra P S,Reddy G B. Advanced glycation end products mediated cellular and molecular events in the pathology of diabetic nephropathy[J]. Biomol Concepts,2016,7(5-6):293-309. doi: 10.1515/bmc-2016-0021 [19] Khalid M,Petroianu G,Adem A. Advanced glycation end products and diabetes mellitus: Mechanisms and perspectives[J]. Biomolecules,2022,12(4):1-17. [20] Sharma R,Sharma M,Reddy S,et al. Chronically increased intrarenal angiotensin II causes nephropathy in an animal model of type 2 diabetes[J]. Front Biosci,2006,1(11):968-976. [21] Zhu Y,Cui H,Lv J,et al. Angiotensin II triggers RIPK3-MLKL-mediated necroptosis by activating the Fas/FasL signaling pathway in renal tubular cells[J]. PloS one,2020,15(3):1-19. [22] Vallon V. The proximal tubule in the pathophysiology of the diabetic kidney[J]. Am J Physiol Regul Integr Comp Physiol,2011,300(5):R1009-R1022. doi: 10.1152/ajpregu.00809.2010 [23] Thomas M C,Burns W C,Cooper M E. Tubular changes in early diabetic nephropathy[J]. Adv Chronic Kidney Dis,2005,12(2):177-186. doi: 10.1053/j.ackd.2005.01.008 [24] Hashimoto Y,Yamagishi S,Mizukami H,et al. Polyol pathway and diabetic nephropathy revisited: Early tubular cell changes and glomerulopathy in diabetic mice overexpressing human aldose reductase[J]. J Diabetes Investig,2011,2(2):111-122. doi: 10.1111/j.2040-1124.2010.00071.x [25] Najafian B, Crosson J T, Kim Y, et al. Glomerulotubular junction abnormalities are associated with proteinuria in type 1 diabetes[J]. J Am Soc Nephrol, 2006, 17 (4 Suppl 2): S53-S60. [26] White K E,Marshall S M,Bilous R W. Prevalence of atubular glomeruli in type 2 diabetic patients with nephropathy[J]. Nephrol Dial Transplant,2008,23(11):3539-3545. doi: 10.1093/ndt/gfn351 [27] Vallon V,Thomson S C. Renal function in diabetic disease models: The tubular system in the pathophysiology of the diabetic kidney[J]. Annu Rev Physiol,2012,74(11):351-375. [28] Tang S C W,Leung J C K,Lai K N. Diabetic tubulopathy: An emerging entity[J]. Contrib Nephrol,2011,170(6):124-134. [29] Tang S C W,Yiu W H. Innate immunity in diabetic kidney disease[J]. Nat Rev Nephrol,2020,16(4):206-222. doi: 10.1038/s41581-019-0234-4 [30] Flyvbjerg A. The role of the complement system in diabetic nephropathy[J]. Nat Rev Nephrol,2017,13(5):311-318. doi: 10.1038/nrneph.2017.31 [31] Zhou W,Marsh J E,Sacks S H. Intrarenal synthesis of complement[J]. Kidney Int,2001,59(4):1227-1235. doi: 10.1046/j.1523-1755.2001.0590041227.x [32] Wada T,Nangaku M. Novel roles of complement in renal diseases and their therapeutic consequences[J]. Kidney Int,2013,84(3):441-450. doi: 10.1038/ki.2013.134 [33] Afshar-Kharghan V. The role of the complement system in cancer[J]. J Clin Invest,2017,127(3):780-789. doi: 10.1172/JCI90962 [34] Braun M C,Reins R Y,Li T B,et al. Renal expression of the C3a receptor and functional responses of primary human proximal tubular epithelial cells[J]. J Immunol,2004,173(6):4190-4196. doi: 10.4049/jimmunol.173.6.4190 [35] de Vries B,Kohl J,Leclercq W K,et al. Complement factor C5a mediates renal ischemia-reperfusion injury independent from neutrophils[J]. J Immunol,2003,170(7):3883-3889. doi: 10.4049/jimmunol.170.7.3883 [36] Liu F,Gou R,Huang J,et al. Effect of anaphylatoxin C3a,C5a on the tubular epithelial-myofibroblast transdifferentiation in vitro[J]. Chin Med J (Engl),2011,124(23):4039-4045. [37] Woroniecka K I,Park A S,Mohtat D,et al. Transcriptome analysis of human diabetic kidney disease[J]. Diabetes,2011,60(9):2354-2369. doi: 10.2337/db10-1181 [38] Zheng J M,Jiang Z H,Chen D J,et al. Pathological significance of urinary complement activation in diabetic nephropathy: A full view from the development of the disease[J]. J Diabetes Investig,2019,10(3):738-744. doi: 10.1111/jdi.12934 [39] Hansen T K. Mannose-binding lectin (MBL) and vascular complications in diabetes[J]. Horm Metab Res,2005,37(Suppl 1):95-98. [40] Li X Q,Chang D Y,Chen M,et al. Complement activation in patients with diabetic nephropathy[J]. Diabetes Metab,2019,45(3):248-253. doi: 10.1016/j.diabet.2018.04.001 [41] Cai K,Ma Y,Wang J,et al. Mannose-binding lectin activation is associated with the progression of diabetic nephropathy in type 2 diabetes mellitus patients[J]. Ann Transl Med,2020,8(21):1-11. [42] Huang Y,Xu J,Wu X,et al. High expression of complement components in the kidneys of type 2 diabetic rats with diabetic nephropathy[J]. Front Endocrinol (Lausanne),2019,10(7):1-9. [43] Mise K,Hoshino J,Ueno T,et al. Prognostic value of tubulointerstitial lesions,urinary N-Acetyl-beta-d-glucosaminidase,and urinary beta2-microglobulin in patients with type 2 diabetes and biopsy-proven diabetic nephropathy[J]. Clin J Am Soc Nephrol,2016,11(4):593-601. doi: 10.2215/CJN.04980515 [44] Han W K,Bailly V,Abichandani R,et al. Kidney injury molecule-1 (KIM-1): A novel biomarker for human renal proximal tubule injury[J]. Kidney Int,2002,62(1):237-244. doi: 10.1046/j.1523-1755.2002.00433.x [45] Adachi T,Arito M,Suematsu N,et al. Roles of layilin in TNF-alpha-induced epithelial-mesenchymal transformation of renal tubular epithelial cells[J]. Biochem Biophys Res Commun,2015,467(1):63-69. doi: 10.1016/j.bbrc.2015.09.121 [46] Limonte C P,Valo E,Drel V,et al. Urinary proteomics identifies cathepsin D as a biomarker of rapid eGFR decline in type 1 diabetes[J]. Diabetes Care,2022,45(6):1416-1427. doi: 10.2337/dc21-2204 [47] Martin-Granado A,Vazquez-Moncholi C,Luis-Yanes M I,et al. Determination of clara cell protein urinary elimination as a marker of tubular dysfunction[J]. Pediatr Nephrol,2009,24(4):747-752. doi: 10.1007/s00467-008-1078-5 [48] Hibi Y,Uemura O,Nagai T,et al. The ratios of urinary beta2-microglobulin and NAG to creatinine vary with age in children[J]. Pediatr Int,2015,57(1):79-84. doi: 10.1111/ped.12470 [49] Nielsen S E,Sugaya T,Tarnow L,et al. Tubular and glomerular injury in diabetes and the impact of ACE inhibition[J]. Diabetes Care,2009,32(9):1684-1688. doi: 10.2337/dc09-0429 [50] Nielsen S E,Sugaya T,Hovind P,et al. Urinary liver-type fatty acid-binding protein predicts progression to nephropathy in type 1 diabetic patients[J]. Diabetes Care,2010,33(6):1320-1324. doi: 10.2337/dc09-2242 [51] Araki S,Haneda M,Koya D,et al. Predictive effects of urinary liver-type fatty acid-binding protein for deteriorating renal function and incidence of cardiovascular disease in type 2 diabetic patients without advanced nephropathy[J]. Diabetes Care,2013,36(5):1248-1253. doi: 10.2337/dc12-1298 [52] Hong C Y,Chia K S. Markers of diabetic nephropathy[J]. J Diabetes Complications,1998,12(1):43-60. doi: 10.1016/S1056-8727(97)00045-7 [53] Lee M,Hong N,Lee Y H,et al. Elevated N-acetyl-beta-d-glucosaminidase,a urinary tubular damage marker,is a significant predictor of carotid artery atherosclerosis in type 1 diabetes,independent of albuminuria: A cross-sectional study[J]. J Diabetes Complications,2018,32(8):777-783. doi: 10.1016/j.jdiacomp.2018.05.019 [54] Kim S R,Lee Y H,Lee S G,et al. The renal tubular damage marker urinary N-acetyl-beta-D-glucosaminidase may be more closely associated with early detection of atherosclerosis than the glomerular damage marker albuminuria in patients with type 2 diabetes[J]. Cardiovasc Diabetol,2017,16(1):1-11. doi: 10.1186/s12933-016-0482-6 [55] Bouvet B R,Paparella C V,Arriaga S M,et al. Evaluation of urinary N-acetyl-beta-D-glucosaminidase as a marker of early renal damage in patients with type 2 diabetes mellitus[J]. Arq Bras Endocrinol Metabol,2014,58(8):798-801. doi: 10.1590/0004-2730000003010 [56] Yang W,Luo Y,Yang S,et al. Ectopic lipid accumulation: potential role in tubular injury and inflammation in diabetic kidney disease[J]. Clin Sci (Lond),2018,132(22):2407-2422. doi: 10.1042/CS20180702 [57] Gala-Bladzinska A,Dumnicka P,Kusnierz-Cabala B,et al. Urinary neutrophil gelatinase-associated lipocalin is complementary to albuminuria in diagnosis of early-stage diabetic kidney disease in type 2 diabetes[J]. Biomed Res Int,2017,2017(8):1-8. [58] Fu W J,Xiong S L,Fang Y G,et al. Urinary tubular biomarkers in short-term type 2 diabetes mellitus patients: A cross-sectional study[J]. Endocrine,2012,41(1):82-88. doi: 10.1007/s12020-011-9509-7 [59] Duan S,Chen J,Wu L,et al. Assessment of urinary NGAL for differential diagnosis and progression of diabetic kidney disease[J]. J Diabetes Complications,2020,34(10):1-8. [60] Tan A L,Sourris K C,Harcourt B E,et al. Disparate effects on renal and oxidative parameters following RAGE deletion,AGE accumulation inhibition,or dietary AGE control in experimental diabetic nephropathy[J]. American Journal of Physiology. Renal Physiology,2010,298(3):F763-F770. doi: 10.1152/ajprenal.00591.2009 [61] Zhao L,Gao H,Lian F,et al. (1)H-NMR-based metabonomic analysis of metabolic profiling in diabetic nephropathy rats induced by streptozotocin[J]. American Journal of Physiology. Renal Physiology,2011,300(4):F947-F956. doi: 10.1152/ajprenal.00551.2010 [62] Blantz R C. Phenotypic characteristics of diabetic kidney involvement[J]. Kidney Int,2014,86(1):7-9. doi: 10.1038/ki.2013.552 [63] Coughlan M T,Nguyen T V,Penfold S A,et al. Mapping time-course mitochondrial adaptations in the kidney in experimental diabetes[J]. Clin Sci (Lond),2016,130(9):711-720. doi: 10.1042/CS20150838 [64] Jiang H,Shao X,Jia S,et al. The mitochondria-targeted metabolic tubular injury in diabetic kidney disease[J]. Cell Physiol Biochem,2019,52(2):156-171. doi: 10.33594/000000011 [65] Kitada M,Kume S,Imaizumi N,et al. Resveratrol improves oxidative stress and protects against diabetic nephropathy through normalization of Mn-SOD dysfunction in AMPK/SIRT1-independent pathway[J]. Diabetes,2011,60(2):634-643. doi: 10.2337/db10-0386 [66] Jha J C,Banal C,Chow B S,et al. Diabetes and kidney disease: Role of oxidative stress[J]. Antioxid Redox Signal,2016,25(12):657-684. doi: 10.1089/ars.2016.6664 [67] Nishikawa T,Edelstein D,Du X L,et al. Normalizing mitochondrial superoxide production blocks three pathways of hyperglycaemic damage[J]. Nature,2000,404(6779):787-790. doi: 10.1038/35008121 [68] Ogura Y,Kitada M,Xu J,et al. CD38 inhibition by apigenin ameliorates mitochondrial oxidative stress through restoration of the intracellular NAD(+)/NADH ratio and Sirt3 activity in renal tubular cells in diabetic rats[J]. Aging (Albany NY),2020,12(12):11325-11336. doi: 10.18632/aging.103410 [69] Jeong H Y,Kang J M,Jun H H,et al. Chloroquine and amodiaquine enhance AMPK phosphorylation and improve mitochondrial fragmentation in diabetic tubulopathy[J]. Sci Rep,2018,8(1):1-13. [70] Zheng X,Narayanan S,Xu C,et al. Repression of hypoxia-inducible factor-1 contributes to increased mitochondrial reactive oxygen species production in diabetes[J]. Elife,2022,11(2):1-22. [71] Liu X,Xu C,Xu L,et al. Empagliflozin improves diabetic renal tubular injury by alleviating mitochondrial fission via AMPK/SP1/PGAM5 pathway[J]. Metabolism,2020,111(10):1-10. 期刊类型引用(1)
1. 杜思娴,刘亚清,殷晓旭,牛浩然,姜峰,龚丽雯. 我国居民抗生素知识掌握情况及影响因素分析. 中国公共卫生. 2024(11): 1318-1325 . 百度学术
其他类型引用(0)
-
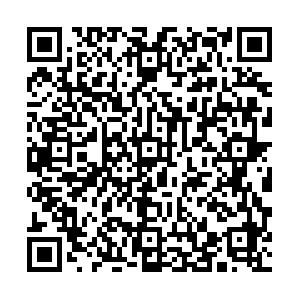
计量
- 文章访问数: 1979
- HTML全文浏览量: 773
- PDF下载量: 50
- 被引次数: 1