The Effects of Caloric Restriction and Intermittent Fasting on Inflammation and Metabolic Diseases
-
摘要: 热量限制(Caloric Restriction,CR)和间歇性禁食(Intermittent Fasting,IF)作为2种饮食干预策略,近年来因其在调节炎症和代谢紊乱中的潜在作用受到广泛关注。随着高热量饮食和久坐生活方式的普及,肥胖、2型糖尿病、心血管疾病等代谢性疾病的发病率显著增加,给公共卫生带来了严峻挑战。本综述探讨了CR和IF通过代谢重塑、自噬激活及抑制炎症通路等机制,在减少炎症、改善代谢健康中的作用。特别是在多发性硬化、系统性红斑狼疮等炎症性疾病中,这2种策略表现出显著的干预效果。此外,CR和IF在肥胖、糖尿病及心血管疾病的代谢调节中也有积极作用。尽管它们在临床应用中具有潜力,但长期依从性和安全性仍是推广的关键挑战。未来研究应侧重个性化干预和多治疗手段的联合应用,以进一步验证CR和IF的临床价值。综上所述,CR和IF为慢性疾病管理提供了新的非药物策略,具有重要的应用前景。Abstract: Caloric restriction (CR) and intermittent fasting (IF) are two dietary intervention strategies that have garnered increasing attention for their potential role in regulating inflammation and metabolic disorders. With the widespread adoption of high-calorie diets and sedentary lifestyles, the incidence of metabolic diseases such as obesity, type 2 diabetes, and cardiovascular diseases has risen significantly, posing major public health challenges. This review explores how CR and IF impact inflammation reduction and metabolic health improvement through mechanisms such as metabolic reprogramming, autophagy activation, and inhibition of inflammatory pathways. Notably, both strategies have shown promising intervention effects in inflammatory diseases like multiple sclerosis and systemic lupus erythematosus. Additionally, CR and IF demonstrate positive effects in the metabolic regulation of obesity, diabetes, and cardiovascular diseases. Despite their clinical potential, long-term adherence and safety remain key challenges for widespread implementation. Future research should focus on personalized interventions and the integration of multiple therapeutic approaches to further validate the clinical value of CR and IF. In summary, CR and IF offer novel, non-pharmacological strategies for managing chronic diseases, presenting significant potential for future clinical application.
-
1. 引言
在现代社会中,饮食结构和生活方式发生了显著变化,特别是高热量饮食和缺乏运动的普遍现象,显著增加了炎症性和代谢性疾病的发病率[1-2]。这些疾病主要包括肥胖、2型糖尿病、心血管疾病、多发性硬化症(Multiple Sclerosis,MS)和系统性红斑狼疮(Systemic Lupus Erythematosus ,SLE)等[1]。这些慢性病不仅严重影响了患者的生活质量,还给全球医疗系统带来了巨大的经济和社会负担[1]。
近年来,随着研究的深入,热量限制(Caloric Restriction,CR)和间歇性禁食(Intermittent Fasting,IF)作为2种非药物的饮食干预手段,因其在改善代谢健康、调节免疫反应以及延缓疾病进展中的潜在作用而受到广泛关注[3−5]。CR通过降低每日热量摄入,不仅促进了代谢平衡的维持,还激活了多种细胞保护机制,如自噬和抗氧化反应;而IF则通过周期性调整进食和禁食时间,促进身体在葡萄糖和酮体代谢之间切换,从而改善整体健康状况[6−8]。大量证据表明,CR和IF能够通过多种机制减轻炎症反应,调节代谢过程,并可能延缓与年龄相关的疾病进程[7,9−11]。这些饮食干预策略不仅在体重管理和血糖控制中显示出显著效果,还表现出调节免疫系统、减少炎症性细胞因子释放等多重益处。在慢性炎症性疾病如MS、SLE以及代谢性疾病如肥胖和糖尿病中,CR和IF已经展现出积极的临床效果,提示其可能成为这些疾病的有效管理工具[7,9−11]。
本综述旨在系统探讨CR和IF在炎症性和代谢性疾病中的具体作用机制、临床应用证据及未来发展方向。通过总结现有研究成果,我们希望为这些饮食干预策略的临床应用和未来研究提供有价值的参考。
2. 热量限制和间歇性禁食的作用机制
2.1 代谢重塑和抗炎机制
CR和IF通过多种代谢调节和抗炎机制,对人体健康产生广泛的积极影响。CR的核心机制在于通过减少每日的热量摄入,触发一系列生理反应,包括降低血糖水平、减少氧化应激以及增强细胞自噬功能[7]。自噬是细胞维持稳态的关键过程,通过清除受损的细胞器和蛋白质,减少炎症反应,并延缓衰老相关的疾病发生[12]。在心脏、肾脏和神经系统中,自噬的增强有助于改善组织功能,抵御病理性损伤[13-14]。例如,CR能够通过腺苷酸活化蛋白激酶(AMP-activated protein kinase,AMPK)的激活,促进心脏和肾脏中的自噬水平,进而减轻缺血/再灌注损伤[13]。在神经系统中,CR和IF通过调节神经肽Y和生长激素受体的活性,刺激自噬,并可能延缓神经退行性疾病的发展[12]。此外,CR和IF能够通过减少炎症相关的生物标志物,改善代谢紊乱,从而对抗慢性炎症[15]。这些机制表明,CR和IF不仅可以促进健康寿命,还能够在延缓代谢疾病和调控炎症方面发挥关键作用。
相比之下,IF的核心机制在于通过周期性禁食触发代谢开关效应,将能量代谢从葡萄糖利用转向脂肪氧化和酮体生成[16]。这一过程中,AMPK和去乙酰化酶1(Sirtuin 1,SIRT1)信号通路被激活,促进脂肪酸氧化、抑制脂肪酸合成并提升线粒体功能[16-17]。酮体(如β-羟基丁酸)不仅提供能量,还通过激活核因子红细胞2相关因子2(Nuclear factor-erythroid factor 2-related factor 2,Nrf2)通路减少氧化应激,并抑制核苷酸结合寡聚结构域样受体蛋白3(nucleotide-binding oligomerization domain-like receptor protein 3,NLRP3)炎性小体,进一步缓解全身炎症[18-19]。此外,IF通过动态激活自噬,上调脑源性神经营养因子,保护神经元并促进再生,可能延缓阿尔茨海默病等神经退行性疾病的进展[16,20-21]。与CR相比,IF在昼夜节律调节中表现更为显著,可能通过优化生物钟基因表达和进食时间进一步改善代谢健康[16]。CR和IF均通过代谢重塑和抗炎机制延缓代谢紊乱相关疾病的发生,并促进健康寿命。然而,IF凭借其快速代谢切换、酮体生成及较高的依从性,展现出更强的灵活性和长期可持续性[16]。
2.2 信号通路的调节
CR和IF对代谢健康和抗炎症反应的调节涉及多个关键信号通路,其中AMPK、SIRT1、哺乳动物雷帕霉素靶蛋白(Mechanistic Target of Rapamycin ,mTOR)和核因子κB(Nuclear factor kappa-light-chain-enhancer of activated B,NF-κB)发挥了重要作用。
AMPK信号通路是细胞能量感应的核心调控因子。CR和IF作为2种有效的代谢干预策略,通过激活AMPK信号通路在调控代谢与炎症反应中发挥重要作用。AMPK由α、β和γ 3个亚基组成,能够感知细胞内ATP水平的变化。当细胞能量水平下降时,AMPK被激活,从而调控细胞能量代谢[22]。激活后的AMPK通过抑制脂肪酸合成、蛋白质合成等能量消耗途径,并促进脂质氧化和葡萄糖代谢,帮助维持能量平衡[22]。在CR和IF条件下,AMPK通过抑制mTORC1信号通路,减少蛋白质合成及细胞内蛋白质积累,进而减轻氧化应激和细胞损伤[23−25]。此外,AMPK还通过抑制NF-κB信号通路,减少促炎性细胞因子如肿瘤坏死因子α(Tumor Necrosis Factor Alpha,TNF-α)和白介素6(Interleukin 6,IL-6)的释放,从而降低全身性炎症[26]。这种调控机制对代谢相关的疾病尤为重要,CR和IF通过AMPK激活能够改善胰岛素敏感性、降低炎症反应并增强代谢健康[26]。
SIRT信号通路在热量限制和禁食中的作用同样重要。SIRT1是依赖于NAD+的去乙酰化酶,它通过去乙酰化作用调节多个代谢和抗炎基因的表达[26−28]。SIRT1通过激活过氧化物酶体增殖物激活受体γ共激活因子1α(Peroxisome Proliferator-Activated Receptor Gamma Coactivator 1 Alpha,PGC-1α)促进线粒体生物合成,并通过抑制NF-κB等促炎途径减少炎症[26,29]。同时,SIRT1与AMPK共同调节代谢稳态,AMPK的激活可以上调NAD+水平,从而进一步激活SIRT1[29]。这种双向调节机制在CR和IF中起到了关键的作用,能够减少细胞内的氧化应激和慢性炎症[29]。
mTOR信号通路则是细胞生长和代谢的核心调节器,CR和IF通过下调mTOR信号通路减少蛋白质合成和细胞生长,从而激活自噬[30-31]。mTOR主要分为两种复合体:mTORC1和mTORC2,mTORC1是自噬的负调控因子,通常在营养丰富的情况下被激活,而在CR和IF期间,由于营养限制,AMPK通过磷酸化结节性硬化症复合体2(tuberous sclerosis complex 2,TSC2)抑制mTORC1,进一步促进自噬[32-33]。通过抑制mTORC1,CR和IF能够有效减少细胞内的蛋白质积累,延缓与衰老相关的代谢紊乱[26,33-34]。
NF-κB信号通路在炎症反应中的作用至关重要。NF-κB是调节炎症基因表达的核心因子,通常通过促炎因子的刺激被激活,如氧化应激和细胞损伤[35]。CR和IF能够通过抑制NF-κB信号通路,减少促炎性细胞因子的释放,如TNF-α和IL-6,从而有效降低系统性炎症[31,36]。此外,CR和IF还通过下调NF-κB的慢性激活,减缓与慢性炎症和神经退行性疾病相关的进程[36-37]。
通过这些信号通路的综合调节,CR和IF能够在多层面上改善代谢健康,减少炎症反应,增强细胞的抗氧化能力,并延缓衰老相关的病理变化。这些信号通路之间的交互作用为CR和IF在预防和治疗代谢性和炎症性疾病中的应用提供了广泛的可能性。
2.3 特殊应用和前景
在预防和治疗代谢疾病和癌症的研究中,CR和IF展现出显著的潜力。研究发现,CR通过代谢重塑和增强自噬,可以选择性抑制癌细胞生长,提升免疫治疗的效果[15]。同时,CR通过改变肠道微生物群,增加有益菌的相对丰度,促进短链脂肪酸的生成,从而改善肠道屏障功能并减少全身性炎症。这些机制不仅有助于维持肠道稳态,还能降低与炎症相关的代谢紊乱风险。同时,IF则通过改善代谢健康和胰岛素敏感性,进一步降低肥胖及代谢性疾病的风险[38]。此外,IF与CR结合的干预策略通过减少脂肪组织中的炎症反应,可能在预防肥胖及相关疾病方面发挥协同作用[39-40]。
总之,CR和IF通过调节关键代谢信号通路,改善自噬水平,抑制促炎反应,在预防衰老相关疾病和代谢紊乱中展现出重要的前景。这些干预策略为未来开发针对代谢和炎症性疾病的治疗手段提供了新的思路和方向[26]。
3. 热量限制和间歇性禁食在炎症性疾病中的应用
3.1 多发性硬化症
MS是1种中枢神经系统的慢性炎症性疾病,其特征是髓鞘的损伤和神经元的退行性变。近年来,CR和IF在动物模型和有限的人类研究中显示出缓解神经炎症的潜力。一项针对MS患者的研究发现,间歇性热量限制在12周内能够改善MS患者的大脑皮层厚度和体积,减少神经炎症的生物标志物,改善神经结构变化[41]。在小鼠模型的研究中,热量限制还被证明可以减少中枢神经系统的髓鞘损伤并促进髓鞘的再生。例如,研究表明,采用模拟禁食饮食能够显著降低实验性自身免疫性脑脊髓炎小鼠模型中的免疫细胞浸润和髓鞘破坏[42]。此外,CR通过调节微胶质细胞的功能,有助于减少髓鞘损伤相关的神经炎症,并促进神经再生[43]。整体来看,CR和IF在MS治疗中的潜力主要体现在它们对神经炎症的调节作用和对神经结构的保护作用,这为MS患者的饮食干预提供了一种新的潜在治疗途径[41−43]。
3.2 系统性红斑狼疮
SLE是一种复杂的自身免疫性疾病,伴有慢性炎症和多器官损伤。近年来,CR和IF在调节SLE相关炎症反应中的潜力逐渐显现。研究表明,IF可有效缓解SLE小鼠模型中的神经认知障碍,减轻全身性炎症,并通过AMPK/PPARγ/NF-κB信号通路减少神经炎症[44]。在人类病例中,个性化饮食干预结合IF被发现能够缓解SLE患者的疲劳、关节疼痛和认知功能障碍[45]。然而,一些研究发现,IF在某些情况下可能加重SLE相关症状,如狼疮性肾炎,提示在应用IF时需要谨慎[46]。同时,CR被认为有助于降低促炎因子的生成,从而减轻SLE的炎症反应并改善代谢健康[47-48]。尽管CR和IF在SLE中的应用展现了潜力,但其效果因个体差异而有所不同,需进一步研究以优化干预策略[45−48]。
3.3 其他炎症性疾病
除MS和SLE外,CR和IF在其他炎症性疾病中的应用也显示出了广阔的前景。炎症性肠病(Inflammatory Bowel Disease,IBD)和类风湿性关节炎(Rheumatoid Arthritis,RA)均为慢性自身免疫疾病,研究表明,饮食干预,如IF和CR,在这些疾病的管理中具有潜在的治疗作用。在IBD中,Th17/Treg细胞的平衡对维持肠道稳态至关重要,饮食调控可以通过代谢途径改善这种平衡[49]。例如,时间限制性进食被证明能够减轻小鼠IBD模型中的肠道炎症[50]。对于RA,研究表明,IF和CR可以通过减少促炎性细胞因子的释放,减轻患者的临床症状[51]。此外,饮食干预还能够降低炎症标志物,改善RA患者的生活质量[52]。
4. 热量限制和间歇性禁食对代谢性疾病的影响
4.1 肥胖与2型糖尿病
CR和IF作为2种不同的饮食干预手段,已被证明对减轻肥胖和控制2型糖尿病(Type 2 Diabetes,T2D)有显著作用。CR通过持续性减少每日热量摄入,能够有效改善胰岛素敏感性、降低体脂和调节葡萄糖代谢[53]。一项研究发现,肥胖患者在经历16周的热量限制干预后,皮下脂肪和内脏脂肪显著减少,胰岛素敏感性得到提升[54]。相比之下,IF则通过周期性减少热量摄入(如交替禁食或5∶2饮食法),对肥胖和T2D的改善也有显著效果[55]。研究表明,IF不仅能够有效减轻体重,还可以通过减少内脏脂肪来改善胰岛素抵抗[56]。在一项综述中,IF被证明能显著降低体重指数(BMI)和脂肪质量,同时改善糖代谢标志物[57]。
虽然CR和IF在短期内均表现出显著的代谢改善效果,但在长期管理方面,2者的作用可能存在差异。研究指出,CR在维持瘦体质量和减少内脏脂肪的长期效果更为显著,而IF尽管在短期内有较好的减重效果,其长期影响尚需更多研究来验证[58-59]。此外,CR能够通过减少内脏脂肪和改善胰岛素敏感性,帮助预防T2D的并发症[60]。
总之,CR和IF均在肥胖和T2D的管理中展示了良好的应用前景。两者通过减少体脂、改善胰岛素敏感性和调节代谢功能,为肥胖和T2D患者提供了有效的非药物治疗选择[61]。
4.2 心血管疾病
CR和IF作为减重和代谢调节的干预手段,对心血管疾病的预防和管理逐渐受到重视。研究表明,CR和IF均能通过多种机制改善心血管健康,尤其在体重管理、血压调控和炎症水平降低等方面具有重要作用[62-63]。首先,间歇性禁食已被证明能够降低体重,并显著改善血脂水平,包括降低低密度脂蛋白和甘油三酯,进而减少动脉粥样硬化风险[62]。此外,一项系统性评估指出,IF对血压的调节效果显著,尤其是在高血压患者中,通过减少每日的卡路里摄入,血压可显著下降,从而降低心血管疾病的风险[63]。
相比之下,热量限制则通过持续的能量摄入减少,有效改善代谢参数。研究显示,CR不仅能够减少体脂,还能够降低内脏脂肪,从而减轻心血管负担[64]。与IF不同,CR的作用更为温和且持续,通过长期的卡路里减少,心血管健康改善效果显著。这些改善主要体现在血糖、血脂以及血压水平的稳定性上[61]。虽然2者在具体机制上略有不同,但研究一致表明,CR和IF在改善心血管健康方面均有显著效果[64-65]。然而,仍需更多长期临床研究来确定这些干预手段在不同人群中的长期安全性和有效性,特别是在心血管高风险患者中[66]。
整体而言,CR和IF作为非药物干预策略,为心血管疾病的预防和管理提供了潜在的新路径。其在体重控制、代谢改善及炎症反应调节中的作用尤为显著,为心血管健康的长期维护奠定了基础[61,66]。
5. 热量限制与间歇性禁食对肠道微生物群及代谢健康的调节作用
近年来,越来越多的研究开始关注CR和IF对肠道微生物群的调节作用及其对代谢健康的潜在影响。热量限制已被证明能够通过改变肠道微生物群的组成,增加有益菌的丰度并减少与代谢紊乱相关的有害菌,从而改善代谢功能[67]。例如,1项动物实验表明,热量限制能够通过增加有益的短链脂肪酸的产生来改善宿主的代谢功能[68]。此外,CR还能够通过调节微生物群的代谢途径来影响能量代谢,并在某些情况下减少代谢相关的疾病风险[69]。尽管这些研究提供了CR与肠道微生物群调节之间的潜在联系,关于具体机制及其对人类健康的长期影响仍需进一步研究。
同样,IF也显示出通过调节肠道微生物群影响宿主代谢健康的潜力。一些研究表明,IF不仅能够通过增加益生菌的丰度来重塑肠道微生物群,还能够促进肠道屏障功能的改善[38]。此外,IF的代谢效应可能还与其对肠道菌群代谢产物的调控有关,这有助于提高宿主的胰岛素敏感性和能量平衡[70]。总结来说,CR和IF通过调节肠道微生物群的组成和代谢产物,展现了其在改善代谢健康方面的潜力。这些研究为代谢性疾病的预防和管理提供了新的思路,但在人类中的临床应用仍需要更多的验证研究。
6. 热量限制和间歇性禁食的临床研究及挑战
近年来,CR和IF在改善代谢健康和管理慢性疾病中的潜力引起了广泛的临床研究关注。尽管动物实验和部分人类研究显示了这些干预的积极效果,但它们在实际临床应用中的推广仍然面临挑战。在代谢性疾病管理中,CR和IF已被证明能够改善胰岛素敏感性、降低体重,并减少炎症标志物。例如,一项针对代谢综合征患者的研究表明,IF能够通过改善肠道微生物群组成,减少全身性炎症,同时促进体重减轻[70]。类似地,CR已在肥胖和T2D患者中被证明能够有效降低体重、改善代谢功能,并降低相关疾病的风险[69]。尽管如此,CR和IF的长期效果仍有待更多研究来验证。现有研究显示,个体对这些干预的反应差异较大,部分研究参与者在长期CR或IF过程中经历了疲劳、情绪波动、能量不足等副作用,这可能影响患者对干预的依从性[38]。此外,老年人或患有慢性疾病的人群在接受CR或IF时的安全性也是1个重要关注点,这些群体是否能够长期安全地坚持这些干预仍需要进一步研究[1]。另1项关键挑战是如何在现代社会的生活方式中保持这些干预的可持续性。由于现代饮食习惯和社会环境的限制,长期维持热量限制或间歇性禁食的饮食方式对于许多人来说可能难以实施。研究者们正在探索更灵活、个性化的饮食方案,以确保人们能够在不显著影响生活质量的情况下获得健康益处[67]。
热量限制和间歇性禁食作为一种改善代谢健康和预防慢性疾病的非药物干预方式,在动物实验和部分临床研究中展示了显著的潜力。这些饮食干预通过调节肠道微生物群、减少炎症、改善胰岛素敏感性等机制,为代谢性疾病的管理提供了新思路。
CR通过长期减少能量摄入,激活AMPK和SIRT1等信号通路,减少氧化应激并抑制炎症相关因子的表达,其作用具有积累性特点。而IF则依赖于周期性禁食,通过代谢切换促进脂肪酸氧化和酮体生成,同时优化昼夜节律相关基因的表达。2者均在缓解慢性炎症、改善代谢平衡方面展现出独特优势,但在适用人群和实施方式上具有互补性。尽管已有研究证实了CR和IF的代谢调控作用,但其长期应用效果、安全性及依从性仍需进一步验证。未来研究应关注以下3个方向:(1)个性化实施方案:针对不同人群(如肥胖、糖尿病和神经退行性疾病患者)探索CR和IF的最优模式。(2)综合干预策略:将饮食干预与运动、心理调节等结合,开发更全面的健康管理工具。(3)现代化实施途径:研究如何将CR和IF整合到现代生活方式中,确保其长效性和可行性。如果这些挑战能够被有效解决,CR和IF将有望作为重要的非药物手段,为代谢疾病的防治提供更广泛的应用前景。
-
[1] Christ A,Lauterbach M,Latz E. Western Diet and the Immune System: An Inflammatory Connection[J]. Immunity,2019,51(5):794-811. doi: 10.1016/j.immuni.2019.09.020 [2] Christ A,Latz E. The Western lifestyle has lasting effects on metaflammation[J]. Nat Rev Immunol,2019,19(5):267-268. doi: 10.1038/s41577-019-0156-1 [3] Zhou R H,Wang Q,Hu X M,et al. The influence of fasting and caloric restriction on inflammation levels in humans: A protocol for systematic review and meta analysis[J]. Medicine (Baltimore),2021,100(15):e25509. [4] Brogi S,Tabanelli R,Puca S,et al. Intermittent Fasting: Myths,Fakes and Truth on This Dietary Regimen Approach[J]. Foods,2024,13(13):1-44. [5] Procaccini C,de Candia P,Russo C,et al. Caloric restriction for the immunometabolic control of human health[J]. Cardiovasc Res,2024,119(18):2787-2800. doi: 10.1093/cvr/cvad035 [6] Hoong C,Chua M. SGLT2 Inhibitors as Calorie Restriction Mimetics: Insights on Longevity Pathways and Age-Related Diseases[J]. Endocrinology,2021,162(8):1-20. [7] Kokten T,Hansmannel F,Ndiaye N C,et al. Calorie Restriction as a New Treatment of Inflammatory Diseases[J]. Adv Nutr,2021,12(4):1558-1570. doi: 10.1093/advances/nmaa179 [8] Mattson M P,Longo V D,Harvie M. Impact of intermittent fasting on health and disease processes[J]. Ageing Res Rev,2017,39:46-58. doi: 10.1016/j.arr.2016.10.005 [9] Diab R,Dimachkie L,Zein O,et al. Intermittent Fasting Regulates Metabolic Homeostasis and Improves Cardiovascular Health[J]. Cell Biochem Biophys,2024,82(3):1583-1597. doi: 10.1007/s12013-024-01314-9 [10] Senesi P,Ferrulli A,Luzi L,et al. Diabetes Mellitus and Cardiovascular Diseases: Nutraceutical Interventions Related to Caloric Restriction[J]. Int J Mol Sci,2021,22(15):1-25. [11] Jaramillo A P,Castells J,Ibrahimli S,et al. Time-Restricted Feeding and Intermittent Fasting as Preventive Therapeutics: A Systematic Review of the Literature[J]. Cureus,2023,15(7):e42300. [12] Ferreira-Marques M,Aveleira C A,Carmo-Silva S,et al. Caloric restriction stimulates autophagy in rat cortical neurons through neuropeptide Y and ghrelin receptors activation[J]. Aging (Albany NY),2016,8(7):1470-1484. doi: 10.18632/aging.100996 [13] Lempiainen J,Finckenberg P,Mervaala E E,et al. Caloric restriction ameliorates kidney ischaemia/reperfusion injury through PGC-1alpha-eNOS pathway and enhanced autophagy[J]. Acta Physiol (Oxf),2013,208(4):410-421. doi: 10.1111/apha.12120 [14] Ferreira-Marques M,Carvalho A,Cavadas C,et al. PI3K/AKT/MTOR and ERK1/2-MAPK signaling pathways are involved in autophagy stimulation induced by caloric restriction or caloric restriction mimetics in cortical neurons[J]. Aging (Albany NY),2021,13(6):7872-7882. doi: 10.18632/aging.202805 [15] Eriau E,Paillet J,Kroemer G,et al. Metabolic Reprogramming by Reduced Calorie Intake or Pharmacological Caloric Restriction Mimetics for Improved Cancer Immunotherapy[J]. Cancers (Basel),2021,13(6):1-25. [16] Zhang A,Wang J,Zhao Y,et al. Intermittent fasting,fatty acid metabolism reprogramming,and neuroimmuno microenvironment: mechanisms and application prospects[J]. Front Nutr,2024,11:1485632. doi: 10.3389/fnut.2024.1485632 [17] Sharma A,Anand S K,Singh N,et al. AMP-activated protein kinase: An energy sensor and survival mechanism in the reinstatement of metabolic homeostasis[J]. Exp Cell Res,2023,428(1):113614. doi: 10.1016/j.yexcr.2023.113614 [18] Kolb H,Kempf K,Rohling M,et al. Ketone bodies: from enemy to friend and guardian angel[J]. BMC Med,2021,19(1):1-15. doi: 10.1186/s12916-020-01826-0 [19] Sharma A,Singh A K. Molecular mechanism of caloric restriction mimetics-mediated neuroprotection of age-related neurodegenerative diseases: an emerging therapeutic approach[J]. Biogerontology,2023,24(5):679-708. doi: 10.1007/s10522-023-10045-y [20] Bhoumik S,Kesherwani R,Kumar R,et al. Alternate day fasting and time-restricted feeding may confer similar neuroprotective effects during aging in male rats[J]. Biogerontology,2022,23(6):757-770. doi: 10.1007/s10522-022-09991-w [21] McCarty M F. Nutraceutical and Dietary Strategies for Up-Regulating Macroautophagy[J]. Int J Mol Sci,2022,23(4):1-16. [22] Yan Y,Zhou X E,Xu H E,et al. Structure and Physiological Regulation of AMPK[J]. Int J Mol Sci,2018,19(11):1-15. [23] Browne G J,Finn S G,Proud C G. Stimulation of the AMP-activated protein kinase leads to activation of eukaryotic elongation factor 2 kinase and to its phosphorylation at a novel site,serine 398[J]. J Biol Chem,2004,279(13):12220-12231. doi: 10.1074/jbc.M309773200 [24] Huo Y,Iadevaia V,Yao Z,et al. Stable isotope-labelling analysis of the impact of inhibition of the mammalian target of rapamycin on protein synthesis[J]. Biochem J,2012,444(1):141-151. doi: 10.1042/BJ20112107 [25] Duvel K,Yecies J L,Menon S,et al. Activation of a metabolic gene regulatory network downstream of mTOR complex 1[J]. Mol Cell,2010,39(2):171-183. doi: 10.1016/j.molcel.2010.06.022 [26] Mayor E. Neurotrophic effects of intermittent fasting,calorie restriction and exercise: a review and annotated bibliography[J]. Front Aging,2023,4:1161814. doi: 10.3389/fragi.2023.1161814 [27] Elkhwanky M S,Hakkola J. Extranuclear Sirtuins and Metabolic Stress[J]. Antioxid Redox Signal,2018,28(8):662-676. doi: 10.1089/ars.2017.7270 [28] Fagerli E,Escobar I,Ferrier F J,et al. Sirtuins and cognition: implications for learning and memory in neurological disorders[J]. Front Physiol,2022,13:908689. doi: 10.3389/fphys.2022.908689 [29] Zhang S,Sun S,Wei X,et al. Short-term moderate caloric restriction in a high-fat diet alleviates obesity via AMPK/SIRT1 signaling in white adipocytes and liver[J]. Food Nutr Res,2022,66:1-12. [30] Huang J,Dibble C C,Matsuzaki M,et al. The TSC1-TSC2 complex is required for proper activation of mTOR complex 2[J]. Mol Cell Biol,2008,28(12):4104-4115. doi: 10.1128/MCB.00289-08 [31] Zhao Y,Jia M,Chen W,et al. The neuroprotective effects of intermittent fasting on brain aging and neurodegenerative diseases via regulating mitochondrial function[J]. Free Radic Biol Med,2022,182:206-218. doi: 10.1016/j.freeradbiomed.2022.02.021 [32] Watson K,Baar K. mTOR and the health benefits of exercise[J]. Semin Cell Dev Biol,2014,36:130-139. [33] Sadria M,Layton A T. Interactions among mTORC,AMPK and SIRT: a computational model for cell energy balance and metabolism[J]. Cell Commun Signal,2021,19(1):1-17. doi: 10.1186/s12964-020-00683-x [34] Ersahin T,Tuncbag N,Cetin-Atalay R. The PI3K/AKT/mTOR interactive pathway[J]. Mol Biosyst,2015,11(7):1946-1954. doi: 10.1039/C5MB00101C [35] Liu T,Zhang L,Joo D,et al. NF-kappaB signaling in inflammation[J]. Signal Transduct Target Ther,2017,2:17023. doi: 10.1038/sigtrans.2017.23 [36] Vella L,Caldow M K,Larsen A E,et al. Resistance exercise increases NF-kappaB activity in human skeletal muscle[J]. Am J Physiol Regul Integr Comp Physiol,2012,302(6):R667-R673. doi: 10.1152/ajpregu.00336.2011 [37] Mattson M P,Arumugam T V. Hallmarks of Brain Aging: Adaptive and Pathological Modification by Metabolic States[J]. Cell Metab,2018,27(6):1176-1199. doi: 10.1016/j.cmet.2018.05.011 [38] Li G,Xie C,Lu S,et al. Intermittent Fasting Promotes White Adipose Browning and Decreases Obesity by Shaping the Gut Microbiota[J]. Cell Metab,2017,26(4):672-685. doi: 10.1016/j.cmet.2017.08.019 [39] Bosch D B L,Boguna M,Sanchez A,et al. Sex-Dependent Metabolic Effects in Diet-Induced Obese Rats following Intermittent Fasting Compared with Continuous Food Restriction[J]. Nutrients,2024,16(7):1-16. [40] Baumeier C,Kaiser D,Heeren J,et al. Caloric restriction and intermittent fasting alter hepatic lipid droplet proteome and diacylglycerol species and prevent diabetes in NZO mice[J]. Biochim Biophys Acta,2015,1851(5):566-576. doi: 10.1016/j.bbalip.2015.01.013 [41] Rahmani F,Ghezzi L,Tosti V,et al. Twelve Weeks of Intermittent Caloric Restriction Diet Mitigates Neuroinflammation in Midlife Individuals with Multiple Sclerosis: A Pilot Study with Implications for Prevention of Alzheimer's Disease[J]. J Alzheimers Dis,2023,93(1):263-273. doi: 10.3233/JAD-221007 [42] Bai M,Wang Y,Han R,et al. Intermittent caloric restriction with a modified fasting-mimicking diet ameliorates autoimmunity and promotes recovery in a mouse model of multiple sclerosis[J]. J Nutr Biochem,2021,87:108493. doi: 10.1016/j.jnutbio.2020.108493 [43] Zarini D,Pasbakhsh P,Nekoonam S,et al. Protective Features of Calorie Restriction on Cuprizone-induced Demyelination via Modulating Microglial Phenotype[J]. J Chem Neuroanat,2021,116:102013. doi: 10.1016/j.jchemneu.2021.102013 [44] Feng Y,Qin J,Wang P,et al. Intermittent fasting attenuates cognitive dysfunction and systemic disease activity in mice with neuropsychiatric systemic lupus erythematosus[J]. Life Sci,2024,355:122999. doi: 10.1016/j.lfs.2024.122999 [45] Kielmann J,Pucci L,Xydis A. Personalized Nutrition and Lifestyle Interventions in Systemic Lupus Erythematosus: A Case Report[J]. Integr Med (Encinitas),2023,21(6):22-27. [46] Hong S M,Lee J,Jang S G,et al. Intermittent Fasting Aggravates Lupus Nephritis through Increasing Survival and Autophagy of Antibody Secreting Cells in MRL/lpr Mice[J]. Int J Mol Sci,2020,21(22):1-12. [47] Constantin M M,Nita I E,Olteanu R,et al. Significance and impact of dietary factors on systemic lupus erythematosus pathogenesis[J]. Exp Ther Med,2019,17(2):1085-1090. [48] Barati M,Ghahremani A,Namdar A H. Intermittent fasting: A promising dietary intervention for autoimmune diseases[J]. Autoimmun Rev,2023,22(10):103408. doi: 10.1016/j.autrev.2023.103408 [49] Zhang S,Zhong R,Tang S,et al. Metabolic regulation of the Th17/Treg balance in inflammatory bowel disease[J]. Pharmacol Res,2024,203:107184. doi: 10.1016/j.phrs.2024.107184 [50] Song S,Chen L,Bai M,et al. Time-restricted feeding ameliorates dextran sulfate sodium-induced colitis via reducing intestinal inflammation[J]. Front Nutr,2022,9:1043783. doi: 10.3389/fnut.2022.1043783 [51] Ranjbar M,Shab-Bidar S,Rostamian A,et al. The effects of intermittent fasting diet on quality of life,clinical symptoms,inflammation,and oxidative stress in overweight and obese postmenopausal women with rheumatoid arthritis: study protocol of a randomized controlled trial[J]. Trials,2024,25(1):1-12. doi: 10.1186/s13063-023-07887-9 [52] Gunes-Bayir A,Mendes B,Dadak A. The Integral Role of Diets Including Natural Products to Manage Rheumatoid Arthritis: A Narrative Review[J]. Curr Issues Mol Biol,2023,45(7):5373-5388. doi: 10.3390/cimb45070341 [53] Ostendorf D M,Caldwell A E,Zaman A,et al. Comparison of weight loss induced by daily caloric restriction versus intermittent fasting (DRIFT) in individuals with obesity: study protocol for a 52-week randomized clinical trial[J]. Trials,2022,23(1):1-19. doi: 10.1186/s13063-021-05959-2 [54] Catenacci V A,Pan Z,Ostendorf D,et al. A randomized pilot study comparing zero-calorie alternate-day fasting to daily caloric restriction in adults with obesity[J]. Obesity (Silver Spring),2016,24(9):1874-1883. doi: 10.1002/oby.21581 [55] Thomas E A,Zaman A,Sloggett K J,et al. Early time-restricted eating compared with daily caloric restriction: A randomized trial in adults with obesity[J]. Obesity (Silver Spring),2022,30(5):1027-1038. doi: 10.1002/oby.23420 [56] Silverii G A,Cresci B,Benvenuti F,et al. Effectiveness of intermittent fasting for weight loss in individuals with obesity: A meta-analysis of randomized controlled trials[J]. Nutr Metab Cardiovasc Dis,2023,33(8):1481-1489. doi: 10.1016/j.numecd.2023.05.005 [57] Morales-Suarez-Varela M,Collado S E,Peraita-Costa I,et al. Intermittent Fasting and the Possible Benefits in Obesity,Diabetes,and Multiple Sclerosis: A Systematic Review of Randomized Clinical Trials[J]. Nutrients,2021,13(9):1-16. [58] Pratchayasakul W,Arunsak B,Suparan K,et al. Combined caloric restriction and exercise provides greater metabolic and neurocognitive benefits than either as a monotherapy in obesity with or without estrogen deprivation[J]. J Nutr Biochem,2022,110:109125. doi: 10.1016/j.jnutbio.2022.109125 [59] Harris L,Hamilton S,Azevedo L B,et al. Intermittent fasting interventions for treatment of overweight and obesity in adults: a systematic review and meta-analysis[J]. JBI Database System Rev Implement Rep,2018,16(2):507-547. doi: 10.11124/JBISRIR-2016-003248 [60] Zhu S,Surampudi P,Rosharavan B,et al. Intermittent fasting as a nutrition approach against obesity and metabolic disease[J]. Curr Opin Clin Nutr Metab Care,2020,23(6):387-394. doi: 10.1097/MCO.0000000000000694 [61] Silverii G A,Cresci B,Benvenuti F,et al. Effectiveness of intermittent fasting for weight loss in individuals with obesity: A meta-analysis of randomized controlled trials[J]. Nutr Metab Cardiovasc Dis,2023,33(8):1481-1489. doi: 10.1016/j.numecd.2023.05.005 [62] Malinowski B,Zalewska K,Wesierska A,et al. Intermittent Fasting in Cardiovascular Disorders-An Overview[J]. Nutrients,2019,11(3):1-18. [63] Allaf M,Elghazaly H,Mohamed O G,et al. Intermittent fasting for the prevention of cardiovascular disease[J]. Cochrane Database Syst Rev,2021,1(1):D13496. [64] Tinsley G M,Horne B D. Intermittent fasting and cardiovascular disease: current evidence and unresolved questions[J]. Future Cardiol,2018,14(1):47-54. doi: 10.2217/fca-2017-0038 [65] Abdellatif M,Sedej S. Cardiovascular benefits of intermittent fasting[J]. Cardiovasc Res,2020,116(3):e36-e38. doi: 10.1093/cvr/cvaa022 [66] Ozcan M,Abdellatif M,Javaheri A,et al. Risks and Benefits of Intermittent Fasting for the Aging Cardiovascular System[J]. Can J Cardiol,2024,40(8):1445-1457. doi: 10.1016/j.cjca.2024.02.004 [67] Kern L,Kviatcovsky D,He Y,et al. Impact of caloric restriction on the gut microbiota[J]. Curr Opin Microbiol,2023,73:102287. doi: 10.1016/j.mib.2023.102287 [68] Tanca A,Abbondio M,Palomba A,et al. Caloric restriction promotes functional changes involving short-chain fatty acid biosynthesis in the rat gut microbiota[J]. Sci Rep,2018,8(1):14778. doi: 10.1038/s41598-018-33100-y [69] Gong T,Di H,Hu Y,et al. Gut microbiota and metabolites exhibit different profiles after very-low-caloric restriction in patients with type 2 diabetes[J]. Front Endocrinol (Lausanne),2023,14:1289571. [70] Guo Y,Luo S,Ye Y,et al. Intermittent Fasting Improves Cardiometabolic Risk Factors and Alters Gut Microbiota in Metabolic Syndrome Patients[J]. J Clin Endocrinol Metab,2021,106(1):64-79. doi: 10.1210/clinem/dgaa644 期刊类型引用(2)
1. 王丽锦. 官桥镇中小学学生生长发育及营养状况调查报告. 中国医药指南. 2024(06): 103-106 . 百度学术
2. 沈浩能,周琼颖,陈琦. 100例学龄前儿童心脏多普勒超声量化参数与体质量和血压的关系. 影像科学与光化学. 2024(06): 625-629 . 百度学术
其他类型引用(0)
-
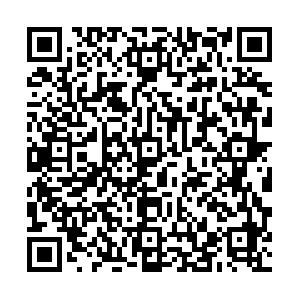
计量
- 文章访问数: 554
- HTML全文浏览量: 155
- PDF下载量: 31
- 被引次数: 2